Ali Awais Amin
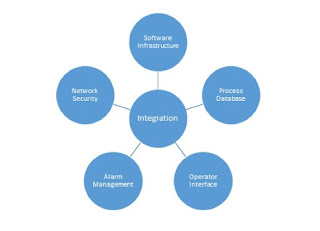
Control system integration is fundamental to industrial
automation. Automation professionals with even a rudimentary knowledge of
system integration realize and understand that isolation is coming to an end. In
fact, control system integrators provide maximum value to the client by
designing the system called for by the project parameters rather than having to
tailor a particular proprietary system according to the specific requirements.
There are five factors involved in integrating different control
systems:
- Software infrastructure
- Process databases
- Operator interface
- Alarm management
- Network security.
Each has a vital role to play. Here is an in-depth look at each
one:
1. Software infrastructure: A modern automation system needs more than just configuration and
monitoring functions. Applications also must be integrated, which is why
software infrastructure is important for system integration. In an open
software architecture employing a client-server scheme, the client application
displays data from a server application.
Developed by the OPC Foundation, the OLE for process
control-unified architecture (OPC-UA) technology is designed to provide simpler
browsing and real-time and historical data exchange. OPC-UA provides
integrators more flexibility to integrate different systems in a desired
configuration instead of being locked into a specific setup by proprietary
technology. The standard's focus is interoperability and is designed to connect
many devices to create a bridge from legacy products to new devices.
2. Process database: Database components primarily include three main components: one
or more tables for the data, a query language (e.g., SQL), and forms for
displaying or entering data. Additional components include customized page
views of data and reporting tools. Moreover, a relational database is a
collection of data items. These data items are organized as a set of formally
described tables from which data can be accessed in many ways without the need
to reorganize the database tables.
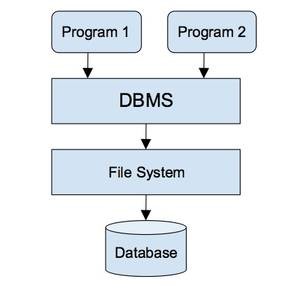
A database management system (DBMS) collects interrelated files
and programs, which allow users to access and modify files. This is an
efficient way to modify, store, and retrieve information. A query language such
as SQL is used to interact with DBMS.
3. Human-machine interface: The human-machine interface (HMI) allows operators to monitor the
state of a control process and issue commands to change the control objective.
In emergency situations, it can also be used to manually override automatic
controls. The primary aspects of HMI configuration are graphics, historical
trend, alarms, reports, and scripts. These capabilities may either be merged
into a single software application or made available as individual components
in a suite.
4. Alarm management: Alarms mark the boundary between normal and abnormal conditions
in the process and serve as the primary means of alerting operators of abnormal
situations in their facilities. Plant operation requires alarms to be
prioritized, relevant, and timely to be effective. Alarm management is critical
when integrating different control systems and so alarm systems need to be
designed to help identify critical issues.
5. Network security: Integrated control and safety systems (ICSS) operate within a
complex environment with organizations increasingly sharing information between
business systems and industrial systems. In addition to this, industrial
systems, which include process control systems, safety systems, and
programmable logic controllers (PLCs) have relied on commercial, off-the-shelf
(COTS) technologies such as Ethernet, TCP/IP and Microsoft Windows for critical
and noncritical functions. However, the isolation from the outside world is
significantly less. In fact, in an event of security breach, the potential loss
of life or production, environmental damage, and compromise to operational
safety are far more serious consequences than loss of trade secrets.
These may have ramifications beyond the targeted organization and
may also damage the infrastructure of the host region or nation.
A detailed cybersecurity analysis and cyber risk assessment
are required. They should include detailed specifications, policies
and procedures for OS patch management, antivirus implementation, and backup
and restore procedures.
Automation does not merely comprise of equipment control. It
includes higher levels of control that manage personnel, materials, and
equipment across all factory production areas. This usually is accomplished
using the documented procedures and software collectively known as the
manufacturing execution system (MES) layer. MES is a control system for managing
and monitoring important tasks in a plant. It supports the planning and control
all the way to finished product, which brings transparency to the highly
complex production tasks.
Four categories of enterprise and control systems are defined by
the ISA-95 depending on their roles. These categories are organized in a
hierarchical model in which activities associated to each category are
specified. The image below illustrates the ISA-95 Functional Hierarchy Model.
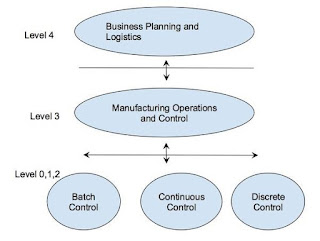
Level 0 defines the actual
physical processes.
Level 1 defines the activities
involved in sensing and manipulating the physical processes.
Level 2 defines the activities
of monitoring and controlling the physical processes. These systems are
typically implemented on PLCs and distributed control systems (DCSs).
Level 3 activities are usually
automated using MES, which also acts as the interface layer between the
control layer (Level 2) and the enterprise resource planning (ERP) layer (Level
4).